Multinary Intermetalloid Clusters
Ternary and quaternary Zintl phases and heterometallic clusters of elemental combinations M/13/15, M/14/15, and M/13/14
Zintl anion chemistry has evolved into a research area encompassing a wide-range of fields from solution based synthetic modification, organometallic, and coordination chemistry to materials science. This significant growth has led to a movement from academic curiosities towards use in potential applications in recent years. The Dehnen Group address compounds based on multinary Zintl anions, in particular, for their fine-tunability of electronic properties and the variability of structural motifs that are inherent to multi-component compounds. In recent years, it has been possible to extend the library of binary Zintl anions and gain access to compounds previously undetermined through careful control and judicious study of reaction conditions.[1]
One particular starting point is the better understanding of the solid mixtures used for the extraction and, thus, isolation of binary anions, especially with regards to the influence of different cations on structures and solubility. In combined experimental and quantum chemical studies it was possible to identify the nature of solid mixtures of A/Tl/Pb (A: Na, K) and K/Tl/Bi that turned out to be extremely complex mixtures of binary and ternary solids like Na17Tl5Pb7, K2TlBi, or K6Tl2Bi3 (Figure 1a).[2] The fusion of K/Ge/As, from which the binary Zintl anion (Ge2As2)2− can be extracted if prepared using a 1:1:1 ratio, in an altered ratio (2:1:2) in a carefully designed two-step synthesis guided by TGA and DSC yielded the novel Zintl phase K2Ge3As3. It is based on oo1{(Ge3As3)2−}, representing an infinite 1-D chain of a complex cluster anion (Figure 1b), and forms bundles of single-crystalline, separable, hair-like metallic fibers (~230 nm in diameter; Figure 1c). This compound shows interesting electronic and optical properties that were subject to detailed experimental and quantum chemical investigations (Figure 1d).[3]
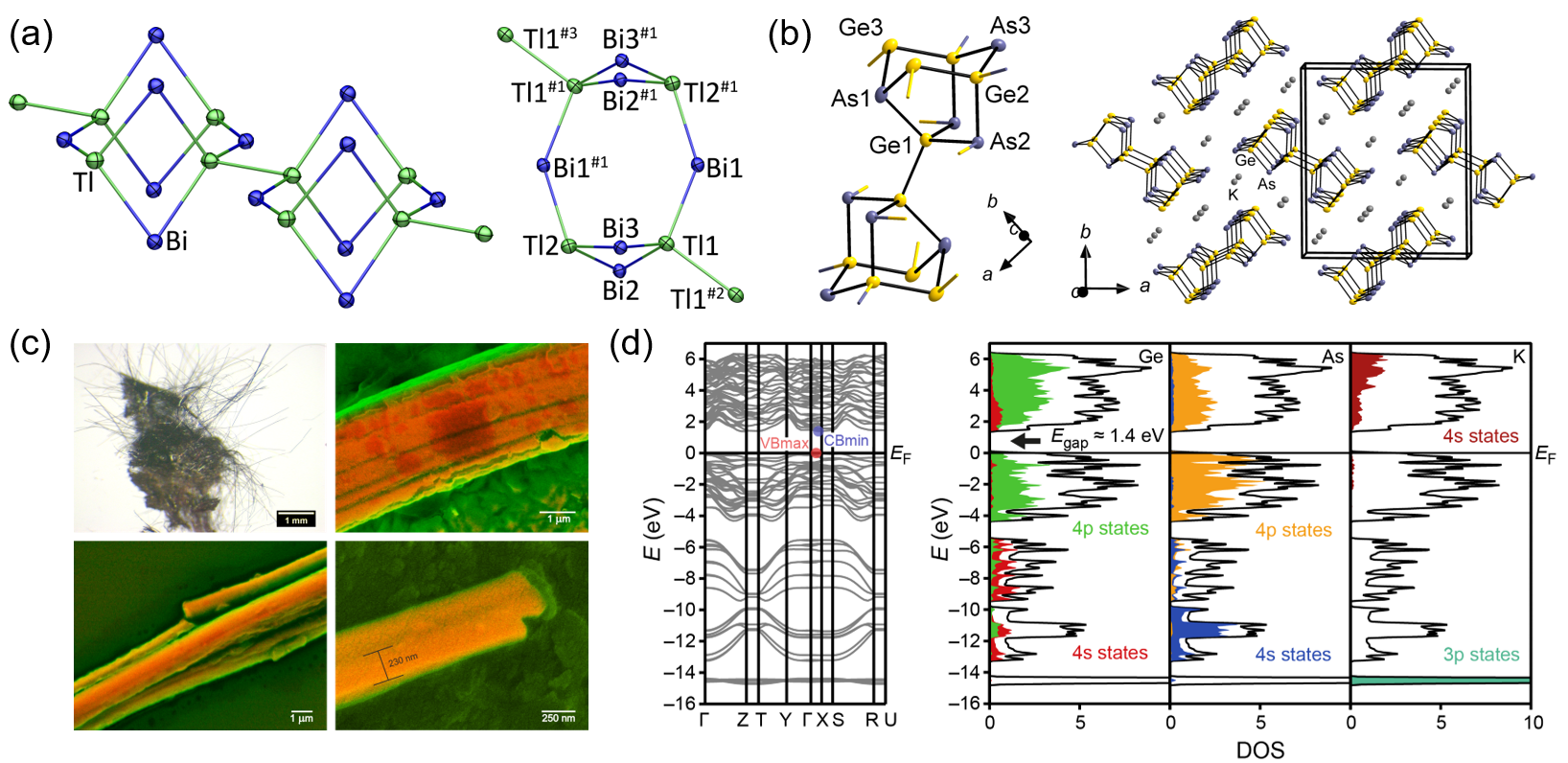
Such multinary solid phases are subsequently extracted (mainly with 1,2-diaminoethane/crypt-222) to obtain salts of binary Zintl anions. In most cases, these anions show an intense mixture of atoms, the correct assignment of which is done by quantum chemistry in combination with mass spectrometry (Figure 2a–c).[4,5] However, the studies also show the limitations of such elemental combinations – thereby revealing other exciting results: mismatch of atomic sizes can provoke an elemental segregation on the molecular scale (and in agreement with immiscibility of the bulk binary solids), such as is observed for the large binary anion (Ge4Bi14)4– (Figure 2d), which is the most Bi-rich main group polyanion known so far.[6,7]
Reactions of the salts of binary Zintl anions with transition metal compounds yield (binary or) ternary multimetallic clusters of the general type [MxE1yE2z]q– (M: transition metal atom; E1: group 13 or 14 atom; E2: group 14 or 15 atom).[1] With the methods developed in the Dehnen Group, it was possible to isolate and study very uncommon multimetallic clusters.[8−12] The cluster anion {[CuSn5Sb2]2}4−, for instance, turned out to be a dimer of heterogeneous superatoms (Figure2e).[8] Regarding the reactivity of these monodisperse, multimetallic species, a combined mass spectrometric and quantum chemical study indicated the potential for O2 activation in the gas phase by means of an Ru/Tl/Bi cluster anion with COD ligands (Figure 2f),[9] and the in-situ formation of polybismuthide anions, also for homoleptic coordination of transition metal ions.[11]
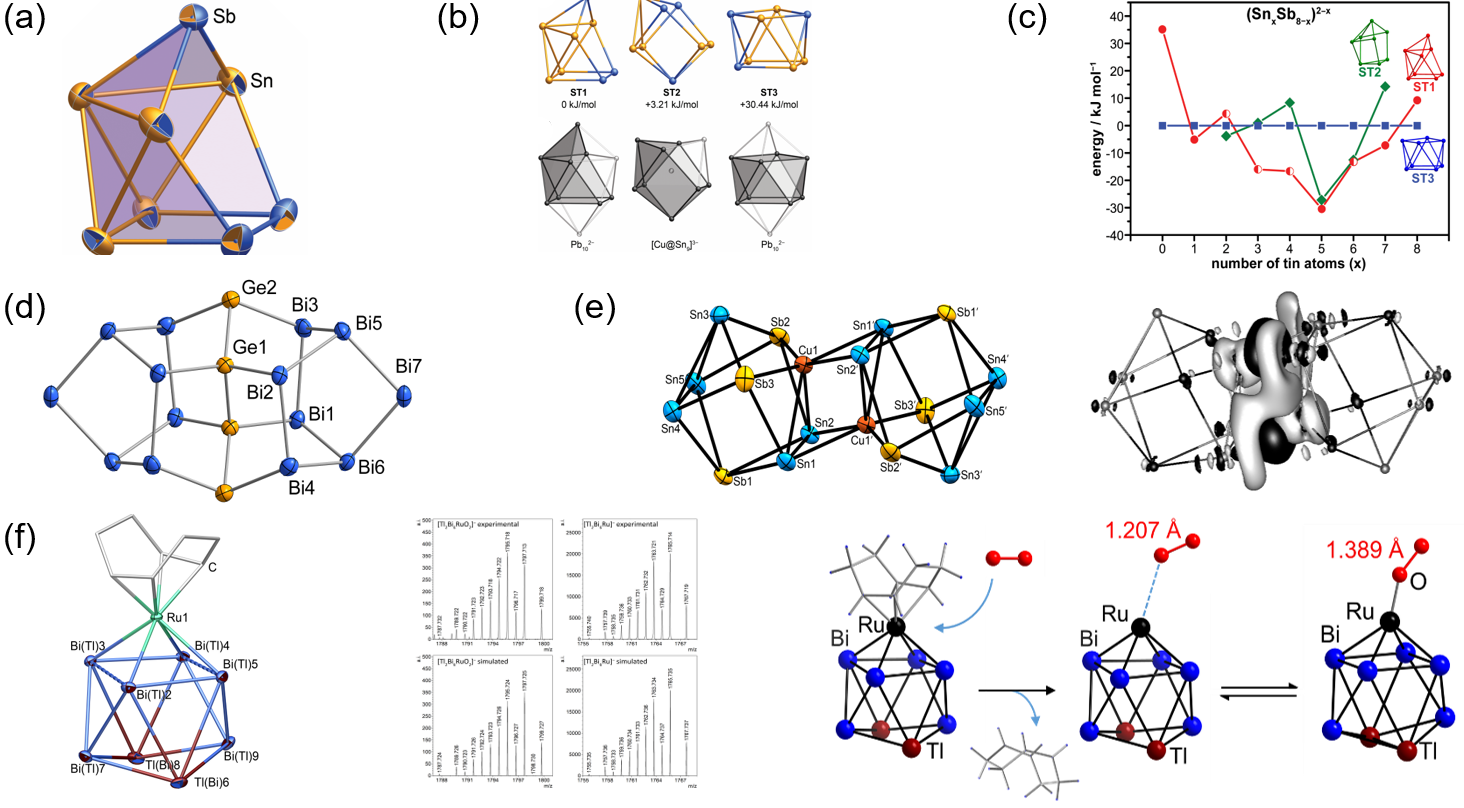
[1] R. J. Wilson, N. Lichtenberger, B. Weinert, S. Dehnen, Chem. Rev. 2019, 119, 8506−8554. [2] N. Lichtenberger, Y. Franzke, W. Massa, F. Weigend, S. Dehnen, Chem. Eur. J. 2018, 24, 12022–12030. [3] A. Eulenstein, D. Bogdanovski, H. Reinhardt, V. Miss, B. Roling, N. Hampp, R. Dronskowski, S. Dehnen, Chem. Mater. 2019, 31, 8839–8849. [4] R. J. Wilson, F. Weigend, S. Dehnen, Angew. Chem. Int. Ed. 2020, 59, 14251‒14255. [5] L. Guggolz, S. Dehnen, Chem. Eur. J. 2020, 26, 11819‒11828. [6] R. J. Wilson, S. Dehnen, Angew. Chem. Int. Ed. 2017, 56, 3098–3102. [7] F. Pan, S. Wei, L. Guggolz, A. Eulenstein, F. Tambornino, S. Dehnen, J. Am. Chem. Soc. 2021, 143, 7176–7188. [8] R. J. Wilson, L. Broeckaert, F. Spitzer, F. Weigend, S. Dehnen, Angew. Chem. Int. Ed. 2016, 55, 11775–11780. [9] N. Lichtenberger, N. Spang, A. Eichhöfer, S. Dehnen, Angew. Chem. Int. Ed. 2017, 56, 13253–13258. [10] R. J. Wilson, F. Hastreiter, K. Reiter, P. Büschelberger, R. Wolf, R. Gschwind, F. Weigend, S. Dehnen, Angew. Chem. Int. Ed. 2018, 57, 15359–15363. [11] N. Lichtenberger, W. Massa, S. Dehnen, Angew. Chem. Int. Ed. 2019, 58, 3222–3226. [12] F. Pan, L. Guggolz, F. Weigend, S. Dehnen, Angew. Chem. Int. Ed. 2020, 59, 16638‒16643.
Intermetalloid clusters embedding f-block ions and other multimetallic clusters of strongly relativistic elements
Multimetallic clusters, in which p-block (semi)metal atoms are combined with d- or f-block metal atoms can generally occur in one of two ways. In the first, the main group atoms form a shell that encapsulates the d- or f-block metal atoms in so-called intermetalloid clusters of the general type [M(x)@E1yE2z]q– (M: d-/f-block metal; E1: group 13 or 14 atom; E2: group 14 or 15 atom). In the second, so-called heterometallic clusters of the general formula [MxE1yE2z]q–, the different (semi)metal atoms are found at equivalent positions within the cluster structures (see also above).[1] Both cluster types serve as molecular models of doped metals or extended intermetallics, which inspires analyses of their electronic and geometric structures and their formation. All of these investigations are thus done in close collaboration of experimentalists with theorists.
Regarding intermetalloid clusters, the Dehnen Group has pioneered methodologies for the introduction of lanthanide ions into such systems,[13−15] which results in unprecedented non-deltahedral cluster motifs. For analyzing the widely unknown and poorly understood formation pathways of such uncommon species, ESI-mass spectrometry and NMR spectroscopy (e.g. 119Sn, 207Pb, 31P, 139La)[4] are used, twinned with comprehensive DFT studies. The group also reported the first clusters with central actinide ions, among them [U@Bi12]3−, for which DFT calculations and magnetic measurements revealed the contribution of f-orbitals to cluster bonding for the first time (Figure 3a−c).[16] Very recent investigations on the corresponding Th4+-centered cluster indicate a strong all-metal 2π-aromaticity with calculated ring currents similar to that in the much more π-electron-rich porphine (Figure 3d).[17] On the example of related cluster architectures embedding group 5 metal atoms, the stepwise formation of such non-deltahedral clusters could be elucidated (Figure 3e).[18] In the course of studying clusters with the heaviest metals, the Dehnen group generally put emphasis on polybismuthide systems and how to grow large examples. In this context, the most Bi-rich multimetallic cluster, and at the same time the largest intermetalloid Znx entity was realized with the cluster anion [K2Zn20Bi16]6− (Figure 3f).[19]
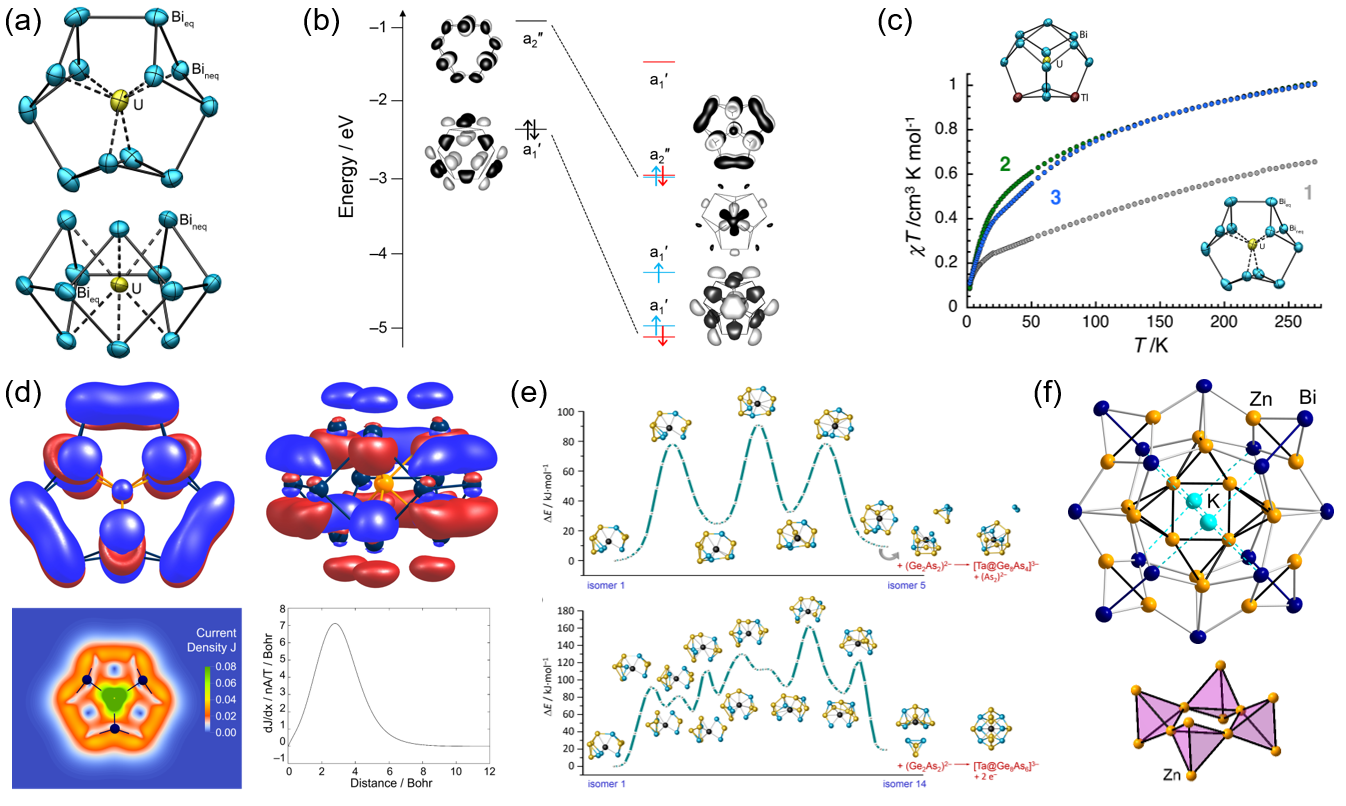
[13] F. Lips, M. Hołyńska, R. Clérac, U. Linne, I. Schellenberg, R. Pöttgen, F. Weigend, S. Dehnen, J. Am. Chem. Soc. 2012, 134, 1181–1191. [14] B. Weinert, F. Müller, K. Harms, S. Dehnen, Angew. Chem. Int. Ed. 2014, 53, 11979–11983. [15] R. Ababei, W. Massa, B. Weinert, P. Pollak, X. Xie, R. Clérac, F. Weigend, S. Dehnen, Chem. Eur. J. 2015, 21, 386–394. [16] N. Lichtenberger, R.J. Wilson, A.R. Eulenstein, W. Massa, R. Clérac, F. Weigend, S. Dehnen, J. Am. Chem. Soc. 2016, 138, 9033–9036. [17] A. R. Eulenstein, N. Lichtenberger, Y. J. Franzke, R. J. Wilson, L. Deubner, F. Kraus, R. Clérac, F. Weigend, S. Dehnen, Nat. Chem. 2021, 13, 149–155. [18] S. Mitzinger, L. Broeckaert, W. Massa, F. Weigend, S. Dehnen, Nat. Commun. 2016, 7, 10480. [19] A. R. Eulenstein, Y. Franzke, P. Bügel, W. Massa, F. Weigend, S. Dehnen, Nat. Commun. 2020, 11, 5122.